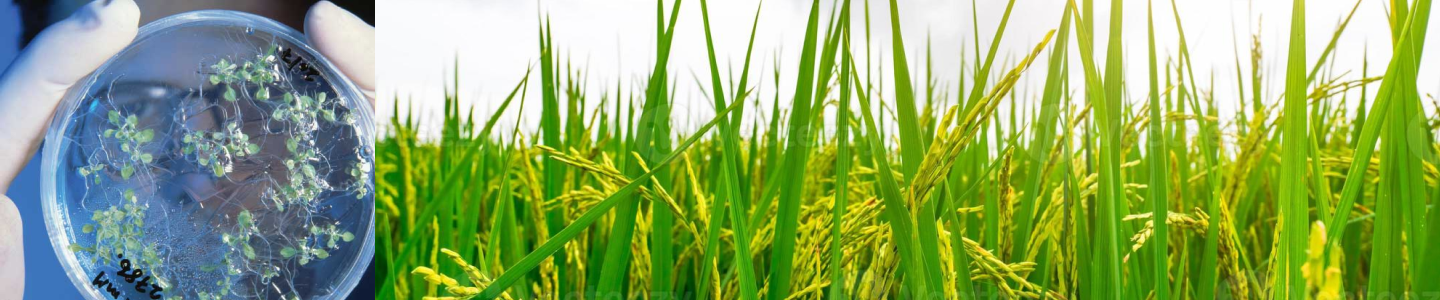
Plants and their associated microbes (collectively known as the microbiota) form functional entities that rely on each other. The microbiota contributes to disease resistance, stress tolerance, and nutrient acquisition in plants. Successful recruitment and maintenance of specific microbial members determine plant-microbiota interactions. Understanding microbiome assembly principles is crucial for sustainable crop production. The plant microbiota can be categorized into three compartments-Rhizosphere: The root-soil interface, Phyllosphere: The leaf surface, Endosphere: Internal tissues.
The host genetics and metabolic processes influence the recruitment of specific microbial taxa in the rhizosphere microbiome. Specific genomic loci, such as Nucleotide-Binding Leucine-Rich-Repeat (NLR) receptors, play a role in shaping the microbiome. Plant root exudates guide rhizosphere microbiome assembly based on the host’s defense and nutritional needs.
While external factors like microbial inocula and climate conditions can alter phyllosphere microbiomes, the plant genotype is now a central focus for engineering plant microbiomes in agriculture. Recent studies highlight the role of defense responses and cell wall integrity in shaping the phyllosphere microbiome.
Mutants in Arabidopsis (such as cuticle mutants and ethylene signaling mutants) exhibit distinct phyllosphere microbiomes compared to wild-type plants. The phosphate starvation response pathway and pattern-triggered immunity also influence the Arabidopsis phyllosphere microbiome. Investigating host genetic effects on microbiome assembly often involves amplicon sequencing of microbial marker genes coupled with genome-wide association studies (GWAS). Several putatively causal genes affecting the relative abundance of plant-associated microbes have been identified. In the Arabidopsis phyllosphere, specific microbial hubs are linked to host genomic loci related to specialized metabolite biosynthesis (e.g., sinapoyl glucose, sinapoyl malate, glucosinolates). While GWAS based on amplicon sequencing has been informative, metagenomic data provides more accurate means for analyzing phyllosphere microbiome compositions. Metagenomics is less prone to over- or underestimating the abundance of specific microbial taxa.
OBJECTIVE OF THE STUDY
The objective of the current study was to investigate how host genetics influence the phyllosphere microbiome (microbes on leaf surfaces) in rice plants.
RESEARCH METHODOLOGY
The researchers used phyllosphere metagenomes from 110 rice accessions and conducted genome-wide association studies (GWAS) to link bacterial abundances with rice genome single nucleotide polymorphisms (SNPs). The study focused on four predominant bacterial orders: Pseudomonadales, Burkholderiales, Enterobacterales, and Xanthomonadales as they were significantly associated with rice genetic variations.
FINDINGS:
-
1. Identification of Lignin Biosynthesis Precursor Gene
The study identified the gene **OsPAL02** as a key player in the biosynthesis of lignin, a crucial component of plant cell walls. OsPAL02 specifically encodes a phenylalanine/tyrosine ammonia-lyase, responsible for the transformation of L-tyrosine into 4-hydroxycinnamic acid (4-HCA), a precursor in lignin production.
2. Effect of OsPAL02 on Phyllosphere Microbiome:
The research explored how variations in OsPAL02 expression influence the composition of the phyllosphere microbiome in rice plants. Deactivation (**OsPAL02-KO**) and overexpression (**OsPAL02-OE**) of OsPAL02 led to significant shifts in the abundance and diversity of microbial communities residing on the plant leaves.
3. Microbiome Community Structure:
The study revealed that Pseudomonadales, Enterobacterales, Sphingomonadales, and other bacterial orders dominated the phyllosphere microbiomes of wild-type (WT) rice plants. The relative abundance of these microbial groups changed significantly in OsPAL02 mutants, indicating a direct link between OsPAL02 and the assembly of specific bacterial communities.
4. Pathway-Associated Enrichment:
Significant enrichment of metabolic pathways, such as Zeatin biosynthesis and Phenylpropanoid biosynthesis, was observed in association with different bacterial orders. Notably, the Phenylpropanoid
biosynthesis pathway, linked to OsPAL02, showed a strong influence on the recruitment and abundance of Pseudomonadales.
5. Impact on Bacterial Blight Incidence:
The research investigated the practical implications of OsPAL02-mediated changes in the phyllosphere microbiome on plant health. Deactivation of OsPAL02 (**OsPAL02-KO**) resulted in increased susceptibility to bacterial blight in rice, with severe disease symptoms observed. Conversely, overexpression (**OsPAL02-OE**) led to enhanced resistance, indicating a direct correlation between OsPAL02, the microbiome, and disease incidence.
6. Metabolite-Associated Insights:
Metabolite analysis revealed that the key metabolite regulated by OsPAL02 is 4-HCA. Changes in OsPAL02 expression altered the concentration of 4-HCA in rice leaves. The study demonstrated that 4-HCA is a critical factor in shaping the phyllosphere microbiome and influencing plant-microbe interactions.
7. Correlation with Rice Genetic Factors:
The research identified natural genetic variations in OsPAL02 between indica and japonica rice varieties. This genetic diversity correlated with differences in the abundance of Pseudomonadales, suggesting a robust connection between OsPAL02, plant genetics, and microbiome assembly.
8. Biotechnological Implications:
The findings have significant implications for biotechnological applications in agriculture. Understanding the role of OsPAL02 in microbiome regulation provides a potential avenue for developing crops with enhanced disease resistance through targeted genetic modifications.
How could this help sustainable agriculture/reduce reliance on pesticides by generating disease-resistant varieties?
-
● Biological Control and Disease Suppression:
Knowledge of the phyllosphere microbiome’s role in disease suppression allows for the identification and selection of specific microbes that naturally protect plants against pathogens. By harnessing these beneficial microbes, scientists can develop crops with enhanced resistance to diseases without the need for chemical pesticides.
-
● Microbiome Engineering for Disease Resistance:
Insights into the genetic factors influencing the composition of plant microbiomes, such as the OsPAL02 gene, provide a foundation for microbiome engineering. Researchers can potentially modify this gene or related pathways to create crops with a microbiome that enhances disease resistance. This targeted approach can help design plants with built-in defenses, reducing the need for external interventions.
-
● Reduced Pesticide Dependency:
Disease-resistant varieties developed through microbiome-informed strategies can mitigate the reliance on chemical pesticides. Farmers can benefit from crops that naturally fend off pathogens, resulting in lower pesticide usage, reduced environmental impact, and decreased health risks associated with pesticide exposure.
-
● Improved Nutrient Cycling and Plant Growth:
Understanding the roles of microbiomes in nutrient cycling and plant growth promotion allows for the selection or engineering of microbes that enhance these processes. This can lead to improved crop yields without the need for excessive chemical fertilizers, promoting sustainable agricultural practices.
-
● Stress Tolerance and Resilience:
Plant microbiomes contribute to stress tolerance, helping crops withstand adverse environmental conditions. Developing varieties with a microbiome that enhances stress resilience can lead to more robust crops capable of thriving in challenging climates, reducing the need for chemical inputs to manage stress-related issues.
-
● Metabolite Production for Defense:
The identification of key metabolites, such as 4-HCA, and their role in shaping the microbiome provides a potential avenue for enhancing plant defenses. Modifying metabolite production through genetic or microbiome engineering can contribute to crops with increased resistance to diseases.
Future Research Avenues:
-
● Microbial Interactions:
Examine intricate dynamics among Pseudomonadales and phyllosphere microbes, revealing collaborations and conflicts.
-
● Functional Metagenomics:
Deploy functional metagenomics to uncover genetic potential and metabolic pathways influencing disease resistance.
-
● Metabolomic Profiling:
Expand metabolomic profiling to various rice varieties, identifying additional metabolites impacting microbiome assembly and disease resistance.
-
● Environmental Impact:
Probe the impact of environmental factors on microbiome functionality, considering soil, climate, and agricultural practices.
-
● Crop-Specific Engineering:
Evaluate transferability of findings to diverse crops, exploring equivalent genes or homologs in different plant species.
-
● Multi-Omics Integration:
Integrate genomics, transcriptomics, metagenomics, and metabolomics for a comprehensive understanding of plant-microbiome interactions.
CONCLUSION
This study provides a direct link between a specific plant metabolite and rice phyllosphere homeostasis opening possibilities for new breeding strategies. This research represents a significant step toward more resilient and eco-friendly crop management by harnessing the power of plant microbiomes. Exploring ways to influence the presence of other beneficial microbes and unlocking additional plant health benefits, can open new avenues for sustainable agriculture.
Leave a Reply